News
Recent articles about ongoing activities and events in the association and in the flagship region
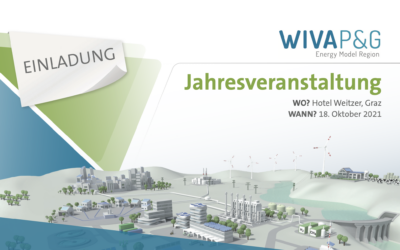
Videos zum Nachsehen der WIVA P&G Jahresveranstaltung 2021 Themenblock 2 „Zukünftige Herausforderung für Wasserstoff & Grüne Gase“/Speicherung von Wasserstoff und Grünen Gasen
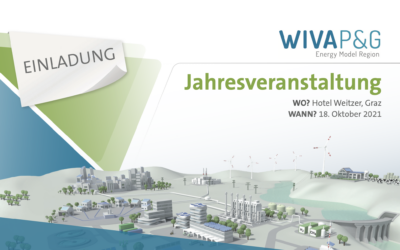
Videos zum Nachsehen der WIVA P&G Jahresveranstaltung 2021 Video 1: Professor Reinhold W. Lang, JKU Linz
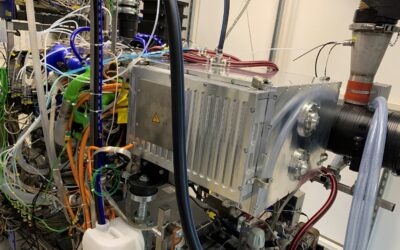
HyTruck – Das Brennstoffzellensystem am AVL Prüfstand
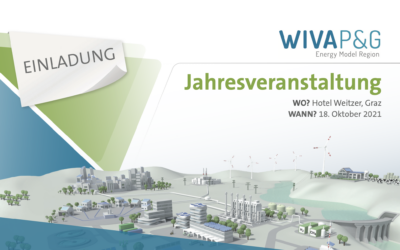
Einladung zur WIVA P&G Jahresveranstaltung 2021 – 18. Oktober, Graz
Presseinformation – Österreichisches Forschungsprojekt „H2Pioneer“ Grüner Wasserstoff für die Halbleiterindustrie
Events
Past Events
October 2024
September 2024
More past events can be found in our >> archives.
Frequently Asked Questions
1. What is hydrogen?
2. How important is hydrogen for our clean energy strategy and long-term sustainability goals?
3. What are the properties of hydrogen?
4. How can hydrogen be produced?
Since hydrogen does not occur naturally in its pure form, it must be produced using energy. To this end, various processes are used that use different primary energy sources and hydrogen compounds, whereby the efficiency and emission of carbon dioxide are important assessment criteria. Hydrogen can be produced from numerous energy sources. The most common are:
- Natural gas (light hydrocarbons) – by steam reforming (SMR)
- Fossil fuel (heavy hydrocarbons) – through partial oxidation
- Coal or biomass – by gasification
- Water splitting – hydrogen production by water splitting by electrolysis using electricity (renewable electricity means renewable hydrogen)
5. What is the blue, green, brown, pink, turquoise and grey hydrogen?
In the energy industry, colour codes or nicknames are used to distinguish the different methods of producing hydrogen. Green hydrogen, blue hydrogen, brown hydrogen and even yellow hydrogen, turquoise hydrogen and pink hydrogen. Hydrogen is a colourless (invisible) gas under normal ambient conditions. Therefore, somewhat confusingly and despite the colourful names, there is no visible difference between the different types of hydrogen. In order to indicate the production method and the energy, often also the raw material, used to produce it, an assignment of colours has been established. However, there is no universal naming convention and these colour definitions can change over time and even from country to country.
- Green hydrogen is produced by water electrolysis using electricity from renewable energy sources, water splitting is an emission-free process. Therefore, green hydrogen is the one produced with very low harmful greenhouse gas emissions. Moreover, green hydrogen can also be produced using biomass for reforming or pyrolysis. In addition to that, several alternatives like direct-solar-water-splitting or biological processes using green algae are in research state.
- Grey hydrogen is produced from natural gas, or methane, using steam methane reformation (SMR) but without capturing the greenhouse gases made in the process. Currently, this is the most common form of hydrogen production.
- Turquoise hydrogen is produced through methane pyrolysis process (thermal splitting of methane), where solid carbon is the byproduct instead of CO2.
- Brown hydrogen is extracted from coal through water gas shift reaction (WGSR), in the last step, where the byproduct of the reaction is CO2. First steps in this process are partial oxidation and gasification.
- Blue hydrogen is grey or brown hydrogen when the carbon generated from the employed process is captured and stored through industrial carbon capture and storage (CCS).
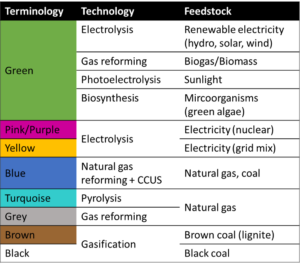
Source: HyCentA
6. How is hydrogen transported?
7. How is hydrogen stored?
Due to the low density of hydrogen, storage and transport with sufficient energy density present technical and economic challenges. Hydrogen storage options can be divided into physical storage methods and chemical storage methods. Physical storage methods are compressed gaseous hydrogen (CGH2), liquid hydrogen (LH2), and cryogenic compressed hydrogen (cCH2): • Gaseous compressed hydrogen at pressures from 300 to 700 bar, CGH2 (compressed gaseous hydrogen) is stored and transported in pressure vessels • Liquid cryogenic hydrogen at temperatures below -252,85 °C (20,3 K) and LH2 (liquid hydrogen) are stored and transported in cryogenic containers Chemical storage methods are liquid hydrides (liquid organic hydrogen carriers, LOHC), solid hydrides (metal hydrides, MH) or ammonia (NH3), predominantly currently in the laboratory stage. Small quantities of hydrogen are usually stored in pressure vessels. Large quantities, for example for seasonal storage of renewable energy, can be stored in underground gas reservoirs. The project Underground Sun Storage 2030 aims for safe, seasonal and large-scale storage of renewable energy in the form of hydrogen in underground gas reservoirs. A pilot plant at a former natural gas reservoir in the municipality of Gampern (Upper Austria) will serve interdisciplinary technical-scientific investigations for the energy future under real conditions until 2025.
8. How does electrolysis work?
Electrolysis is an electrochemical process, what means that electricity is applied in a so-called electrochemical cell leading to the breakdown of molecules in this cell. An electrochemical cell consists of two electrodes (anode and cathode) separated by an ion-conductive material (electrolyte). In the case of water electrolysis, the application of electricity leads to the splitting of water molecules (H2O – liquid or gaseous) to H2 (gaseous) and O2 (gaseous). The two product gases are leaving the cell and can be used separately. Via electrolysis electrical energy can be converted into chemical energy.
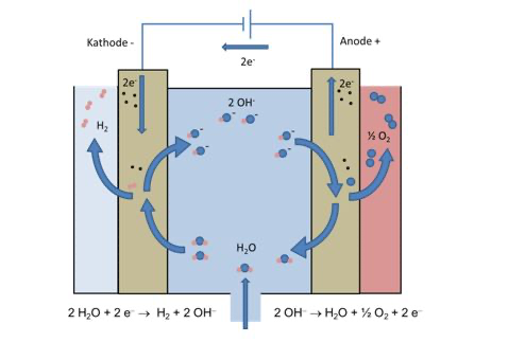
Source: Wasserstoff in der Fahrzeugtechnik, M. Klell, H. Eichlseder, A. Trattner; Springer Vieweg, Wiesbaden, 2018
9. What different types of electrolysers exist?
Electrolysis technologies are typically distinguished according to the used electrolyte and the operating temperature. The alkaline electrolysis (AEL) uses potassium hydroxide as electrolyte and is working at low temperature around 60-70 °C. It is an industrialized technology and several hundreds of MW are installed.
Polymer electrolyte membrane electrolysis (PEMEL) is a technology characterized by the membrane separating the anode and the cathode. This membrane can transport H+-Ions (protons) and serves as electrolyte in the cell. PEM electrolysers are available commercially up to multi-MW scale. High power density, compact design and operation under differential pressure (hydrogen is produced under pressure up to 160 bar) are the main characteristics of PEM electrolysers. However, the technology is expensive and requires critical raw materials, such as platinum.
The alkaline counterpart to PEM electrolysers is the anion exchange membrane electrolyser (AEMEL). It employs diluted potassium hydroxide as electrolyte and an anion-conducting membrane to separate anode and cathode. AEM electrolysers can be operated under differential pressure (30 bar) and can be produced from abundant, low-cost materials. This technology is currently on to commercialization.
Solid oxide electrolysis (SOEL) is a so-called high-temperature technology operating at up to 1000 °C, while all the other electrolyser technologies are low-temperature applications. O2–Ion conducting materials are employed as electrolyte. This technology promises very high efficiency and operation at up to 30 bars. However, so far this technology is still on the level of R&D.
10. What is Power-to-Gas, Power-to-Hydrogen, Power-to-Heat, Power-to-liquid and Power-to-X?
P2G: Renewable electricity (power) is used to create a gaseous fuel. Power-to-Gas (P2G) is the only technology capable of providing storage at terawatt-hour scale. Hydrogen is stored in a storage system like tanks, caverns, or the natural gas grid. Using the natural gas grid would allow for very large amounts of renewable hydrogen to be stored very economically, as very little new infrastructure needs to be built. Power-to-Hydrogen (P2H2) means a technology in which hydrogen is produced via electrolysis process. Hydrogen becomes the carrier of renewable energy when renewable power is used for this process. Power-to-Liquid (P2L) is a process where the products from the P2G process are subsequently converted to liquid fuels, for example by the Fischer-Tropsch process where H2 and CO2 react to for liquid hydrocarbons (petroleum, diesel). Power-to-Heat (P2H) allows the utilization of excess electricity generated by renewable energy sources in the form of heat.
Power-to-X (P2X) is a series of technologies allowing the conversion of electricity into energy carriers, chemicals and heat. A term which regroups different employed techniques in this regard, where X refers to the result of conversion.
Within the scope of Renewable Gasfield a power-to-gas plant is set up in Southern Styria. A PEM-Electrolysis produces green hydrogen from regenerative resources, mainly a 1 .5 MW PV field onsite. H2 is either used for a 350 bar hydrogen filling station or for a methanation process of carbon-rich biogas. In the southern part of Styria (Austria) an existing biogas plant is operating at half capacity. Coupling it with a load-flexible methanation will enable the generation and storage of synthetic natural gas (SNG) in the gas grid.
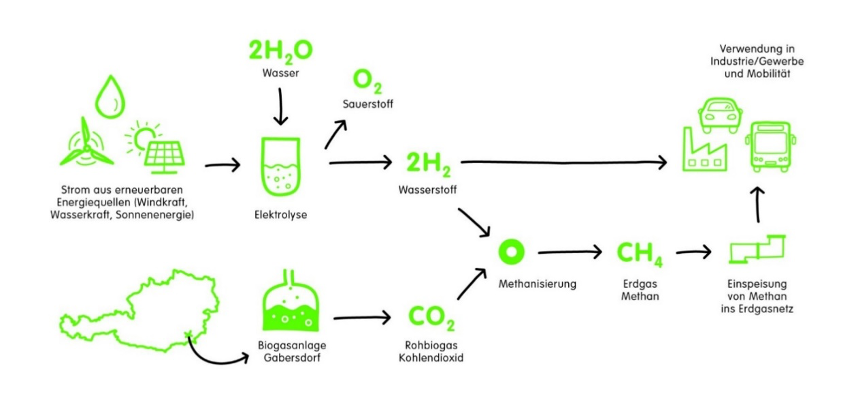
Source: WIVA project Renewable Gasfield
11. How will hydrogen support challenging sectors for green energy, like chemicals, cement and steel?
12. Is it safe to use hydrogen?
13. As energy carriers, what is the difference between electricity and hydrogen?
We support research and development
Renewable hydrogen as
central component
Expertise
WIVA P&G combines experience out of more than 30 completed and running projects
Contact
For all general questions please contact
office@wiva.at
Network
Our industry and research members rely on longstanding eperience in the fields of hydrogen and green energy.